Overcoming Critical Issues in Glioblastoma Multiforme (GBM) Therapeutic Development
Glioblastoma multiforme (GBM) is one of the most common and difficult-to-treat adult brain tumors.1 With an estimated 10,000 new US diagnoses per year2 and a near 100% recurrence rate,3 safe and efficacious brain-penetrant GBM therapies are essential to combat this complex and often deadly disease. However, developing drugs that effectively treat GBM has proved exceedingly challenging to date.
The Current Neurotherapeutic Landscape: Effective GBM Pharmacological Treatments are Few and Far Between
GBM patients are faced with limitations on both the availability and efficacy of FDA-approved therapeutics. The current standard of care — surgical resection, followed by radiation therapy and temozolomide, and temozolomide maintenance therapy — is largely ineffective, with 95% of patients suffering a relapse.4,5
Furthermore, temozolomide (TMZ) has been the only first-line approved drug to treat GBM in the last 20 years, with limited efficacy and a high rate of resistant patients.5 Additionally, only four orphan drugs out of 161 and one implant have been approved for the treatment of GBM between 1983 and 2020:6
GBM Compound | Year of Approval |
---|---|
5-aminolevulinic acid | 2017 |
Bevacizumab | 2009 |
Polifeprosan 20 with carmustine | 2003 |
Lomustine | 1976 |
Temozolomide | 2006 |
Bevacizumab can be used for recurrent GBM, yet its use has not improved overall survival, only surrogate outcomes, such as progression-free survival.4,5 Likewise, carmustine and temozolomide have also been used in the clinical setting, but with discouraging results and no improvement in patient outcomes.6
A Lack of Accurate GBM Preclinical Models is Slowing Development of Better GBM Therapeutics
A major bottleneck in GBM drug development is a lack of preclinical models that accurately predict pathogenesis and resistance. In a perfect world, GBM models would effectively mimic:
- Genetic background of the human tumor7
- Intra-tumoral heterogeneity7
- Tumor microenvironment and blood-brain barrier (BBB)7
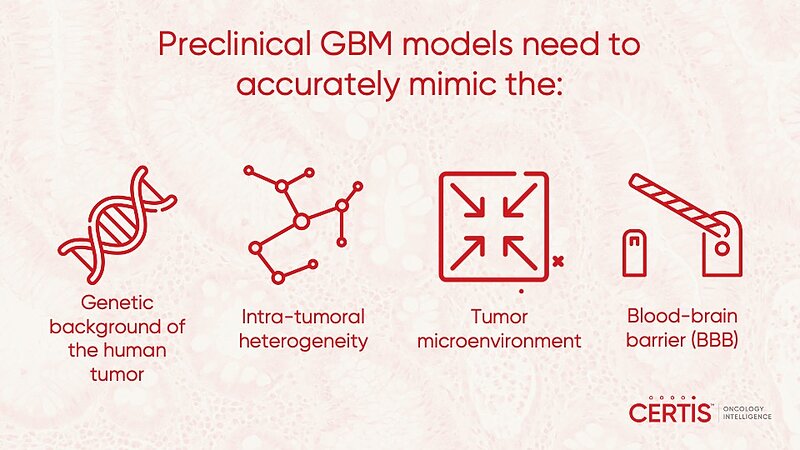
As with any model, reproducibility and cost-effectiveness are important, yet precisely duplicating optimal preclinical models is particularly challenging for GBM drug developers.
Let’s take a closer look at why.
Challenge: An Evolving Genetic Background
GBM can be classified at the genetic level as isocitrate dehydrogenase (IDH) - wildtype (WT), which tends to have a worse prognosis than the other category IDH-mutant.8 At the transcriptional level, there are four subtypes, depending on the mutational status and expression level of a handful of genes, including PDGFR, IDH1, EGFR, and NF1.4,5,9 These subtypes are identified using bulk analysis of 200 tumors and highlight the inter-tumoral heterogeneity of GBM.9 Despite this initial characterization, additional mutations in common oncogenic pathways, including CDKN2A/B, TP53, and PTEN, occur within these subtypes, and the genetic landscape of a GBM tumor is constantly changing over time.5,10 Addressing the ever-evolving genetic landscape of an individual tumor and accounting for the extreme genetic variability across individual patients with a traditional preclinical model can be like trying to hit hundreds of moving targets, all at once.
Challenge: Temporal and Spatial Intra-Tumoral Heterogeneity
More recently, single-cell RNA-seq analysis of five GBM tumors has revealed the extreme intra-tumoral heterogeneity within single GBM tumors.11 The study, by Patel et al. shows that GBM tumors can contain representative cells from each transcriptional subtype, which can play a significant role in treatment resistance. Patients whose tumors were classified as being in the pro-neural subtype (which was thought to be the prognostic indicator with the best clinical outcomes) with markers of other subtypes had poorer survival.10,11
The study by Patel et al. also identifies a spectrum of stemness within GBM tumors, likely driven by the presence of glioblastoma stem-like cells (GSCs).5,11 It is now widely accepted that GSCs exhibit a unique tumor-initiating ability, and aggressive GBM tumors, as well as treatment resistance and relapse, are driven by these cell types.5,11 The extreme heterogeneity has been confirmed by additional single-cell genomics studies.10 In addition, studies by Sorttoriva et al using fluorescence-guided multiple sampling techniques during GBM resection demonstrate the extensive 3D spatial heterogeneity within GBM tumors and confirm that within the same tumor, different subtypes exist.12
Challenge: The Tumor Microenvironment and Blood-Brain Barrier
The genetic and transcriptomic complexity and heterogeneity of GBM tumors are further compounded when looking at the immune cells that make up the microenvironment. For instance, tumor-infiltrating lymphocytes (TILs) are enriched in certain GBM subtypes and depleted in others.13 IDH-WT tumors are also associated with higher TIL infiltration.13 IDH mutational status and GBM transcriptional subtypes also affect the presence of macrophages, neutrophils, and other immune cells within the tumor microenvironment.13 Mimicking this additional layer of heterogeneity presents a further challenge for traditional preclinical models.
On a more macro level, in vitro and most in vivo models fail to mimic the blood-brain barrier (BBB), a major reason why promising drug candidates for GBM have failed.14 Crossing the BBB is a huge challenge for drug development across many CNS indications and 98% of small molecules and 100% of large-molecule therapeutics fail to accumulate at therapeutically relevant concentrations.14
The Future Neurotherapeutic Landscape: A Steadily Growing GBM Therapeutic Pipeline
According to ClinicalTrials.gov, there are currently 327 actively recruiting GBM clinical studies.15
While the GBM drug development pipeline is growing, current patient outcomes remain bleak. Even with the surge of immunotherapies, immune-checkpoint inhibitors have demonstrated limited efficacy in GBM patients.16 The sad reality is that nearly all GBM patients experience tumor progression and universal mortality,17 with an average five-year survival rate of just 7%.2 The inability to develop drugs that significantly improve survival rates in GBM patients highlights the compelling need to revisit current preclinical study designs and prioritize the use of clinically relevant preclinical GBM models and intracranial engraftment techniques that take the blood brain barrier (BBB) and intratumoral heterogeneity into account.
The Future of GBM Drug Discovery: Clinically Relevant Glioblastoma Xenograft Models
Certis Oncology Solutions is dedicated to helping drug developers fulfill the unmet need for safe and efficacious GBM therapeutics. Learn more about how Certis is advancing GBM drug development through well-characterized orthotopic patient-derived xenograft (O-PDX) models that closely recapitulate the clinical characteristics of GBM and account for inter- and intra-tumoral heterogeneity,5,7 as well as specialized intracranial engraftment and dosing techniques.
Reserve Your Virtual Seat for an Upcoming Live Webinar
Learn about innovations in glioblastoma preclinical model development and how to mitigate drug development challenges related to therapies for cancers of the brain. Join Certis Scientist Monika Buczek, PhD, Director of Business Development, for an in-depth discussion on the importance of selecting clinically relevant glioblastoma (GBM) models that closely recapitulate human GBM as well as specialized intracranial engraftment and dosing techniques.
References
- Harder, BG, Biomquist, MR, Wang, J et al. Developments in Blood-Brain Barrier Penetrance and Drug Repurposing for Improved Treatment of Glioblastoma. Front. Oncol. Oct. 23, 2018; Sec: Cancer Molecular Targets and Therapeutics.
- National Brain Tumor Society. Glioblastoma Facts & Figures. Accessed on: June 23, 2022.
- van Linde, M. E., Brahm, C. G., de Witt Hamer, P. C., et al. (2017). Treatment Outcome of Patients with Recurrent Glioblastoma Multiforme: A Retrospective Multicenter Analysis. J Neurooncol. 2017. 135(1):183 – 192.
- Fernandes C, Costa A, Osório L et al. Current Standards of Care in Glioblastoma Therapy. In: De Vleeschouwer S, editor. Glioblastoma [Internet]. Brisbane (AU): Codon Publications; 2017 Sep 27. Ch 11. NIH website.
- Patrizii M, Bartucci M, Pine SR, Sabaawy HE. Utility of Glioblastoma Patient-Derived Orthotopic Xenografts in Drug Discovery and Personalized Therapy. Front Oncol. 2018;8:23. Published 2018 Feb 12.
- Johann P, Lenz D, Ries M. The Drug Development Pipeline for Glioblastoma-A Cross Sectional Assessment of the FDA Orphan Drug Product Designation Database. PLoS One. 2021;16(7):e025292.
- Akter F, Simon B, et al. Preclinical Tumor Models of Primary Brain Tumors: Challenges and Opportunities. Biochim Biophys Acta Rev Cancer. 2021;1875(1):188458.
- Sottoriva A, Spiteri I, Piccirillo SG et al. Intratumor Heterogeneity in Human Glioblastoma Reflects Cancer Evolutionary Dynamics. Proc Natl Acad Sci U S A. 2013;110(10):4009-4014.
- Verhaak RG, Hoadley KA, Purdom E, et al. Integrated genomic analysis identifies clinically relevant subtypes of glioblastoma characterized by abnormalities in PDGFRA, IDH1, EGFR, and NF1. Cancer Cell. 2010;17(1):98-110. doi:10.1016/j.ccr.2009.12.020.
- Qazi MA, Vora P, Venugopal C et al. Intratumoral Heterogeneity: Pathways to Treatment Resistance and Relapse in Human Glioblastoma. Ann Oncol. 2017;28(7):1448-1456.
- Patel AP, Tirosh I, Trombetta JJ et al. Single-Cell RNA-Seq Highlights Intratumoral Heterogeneity in Primary Glioblastoma. Science. 2014;344(6190):1396-1401.
- Sottoriva A, Spiteri I, Piccirillo SG et al. Intratumor Heterogeneity in Human Glioblastoma Reflects Cancer Evolutionary Dynamics. Proc Natl Acad Sci U S A. 2013;110(10):4009-4014.
- Pombo Antunes AR et al. Understanding the Glioblastoma Immune Microenvironment as Basis for the Development of New Immunotherapeutic Strategies. Elife. Feb. 4, 2020;9:e52176.
- Pardridge WM. The Blood-Brain Barrier: Bottleneck in Brain Drug Development. NeuroRx. 2005;2(1):3-14.
- ClinicalTrials.gov. Accessed on August 1, 2022.
- Brahm CG, van Linde ME, Enting RH et al. [The Current Status of Immune Checkpoint Inhibitors in Neuro-Oncology:]((https://pubmed.ncbi.nlm.nih.gov/32143288/) A Systematic Review. Cancers (Basel). Mar. 4, 2020;12(3):586.
- Glioblastoma [Internet]. De Vleeschouwer S, editor. Brisbane (AU): Codon Publications; 2017 Sep 27.