Blood Proteomics: Revealing Clues About Immune Checkpoint Therapy Response
This blog post was written by RayBiotech, a CRO that delivers cutting-edge array technologies, high-performance single protein analysis tools and quality-focused services. Their services are available on the Scientist.com marketplace.
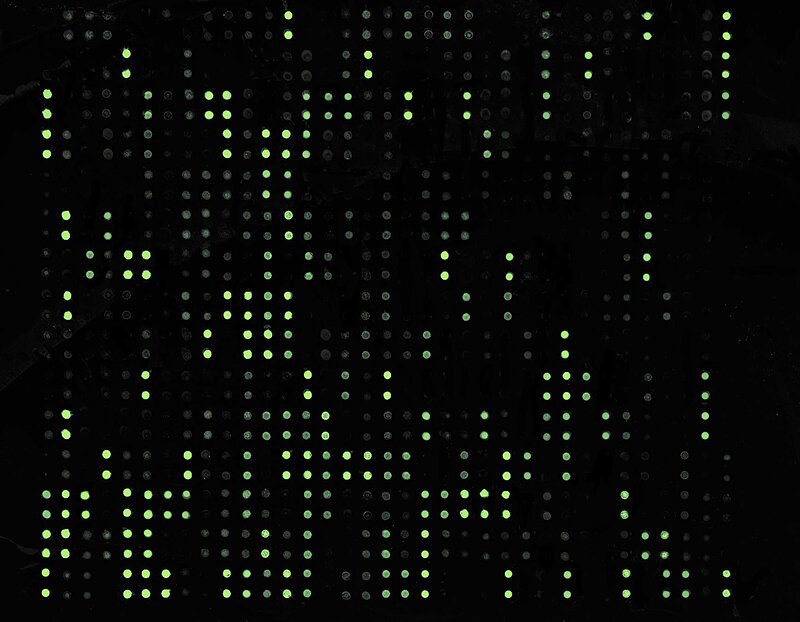
Immune checkpoint inhibitors (ICIs) have transformed treatment for patients with locally advanced or metastatic solid tumors by offering significant survival benefits across several cancer types and have become a cornerstone of cancer therapy alongside surgery, chemotherapy and radiation therapy. These therapies target immune checkpoints — molecules that tumors exploit to suppress immune responses. By blocking these checkpoints, ICIs restore immune activity within the tumor microenvironment (TME), unleashing a more robust antitumor response.
Among these checkpoints, PD-1 and PD-L1 (a receptor on T cells and its ligand expressed on tumor and immune cells, respectively) and CTLA-4 (an immune-regulatory receptor that inhibits T cell activation) have emerged as critical therapeutic targets. Currently, six ICIs targeting these molecules such as pembrolizumab and nivolumab, are FDA-approved as first- and second-line treatments for malignancies, including non-small cell lung cancer (NSCLC), melanoma and renal cell carcinoma. Despite these advancements, response rates remain limited; in NSCLC, for example, approximately 48-58% of patients respond to ICI-chemotherapy combinations, while 27-46% respond to ICI monotherapy. This highlights a substantial gap, as many patients do not experience significant clinical benefits from ICIs. Efficacy is often limited by primary resistance — where patients fail to respond initially — and adaptive resistance, which develops over time. TME factors, such as cytokines, immunosuppressive cells and checkpoint protein expression, drive resistance mechanisms, influence response variability and contribute to immune-related adverse events, some of which can be severe.1 These challenges highlight the urgent need for predictive biomarkers to better identify responders, optimize patient selection and minimize risks.
Tissue vs. Blood Biomarkers
Current clinical practice relies on tumor tissue-based biomarkers, such as PD-L1 expression, tumor mutational burden (TMB; the number of mutations in tumor DNA that increase neoantigen presentation), microsatellite instability (MSI; reflecting DNA repair defects) and immune-related gene expression profiling. However, these biomarkers have significant limitations. Biopsies are invasive, challenging to obtain and may be further complicated by a patient’s condition, potentially delaying treatment decisions. Additionally, single biopsies often fail to capture the spatial heterogeneity and dynamic immune interactions of the TME, offering only a limited snapshot of the tumor’s immune landscape.4
These challenges have led to an increased interest in blood-based biomarkers. Non-invasive and accessible, they allow real-time monitoring of systemic immune responses, making them suitable for continuous assessment during ICI treatment. The effectiveness of ICIs is largely shaped by TME cytokines, which either promote immune activation or create an immunosuppressive environment that hinders efficacy. For instance, elevated levels of interleukin-6 (IL-6) are associated with ICI resistance, as IL-6 enhances regulatory T cell activity and inhibits cytotoxic T cell function. Similarly, transforming growth factor-beta (TGF-β) contributes to immune suppression by inhibiting the proliferation and activation of effector T cells. Conversely, higher concentrations of interferon-gamma (IFN-γ) are linked to favorable responses, reflecting a more active anti-tumor immune response. Tumor necrosis factor-alpha (TNF-α) has also been implicated in modulating ICI efficacy; studies suggest TNF-α can upregulate PD-L1 expression on tumor cells, potentially leading to adaptive resistance.2 Profiling these cytokines and their interactions with immune cells provides valuable insights into how the TME modulates therapy response and resistance.
To capture the complexity of immune interactions, multi-analyte profiling through multiplexed antibody array technology enables the simultaneous detection of multiple cytokines and immune markers, including those at low abundance, with high sensitivity and specificity. Antibody arrays are adaptable to various sample types, such as blood plasma, tumor lysates and TME-specific fluids, making them valuable for tailoring ICI therapy, refining patient selection and advancing precision medicine in immuno-oncology.4,5
Recent studies using multiplex protein detection in blood have identified immune signatures that predict patient response to ICI therapy. By profiling multiple immune-related proteins, these studies reveal specific protein signatures that distinguish responders from non-responders, underscoring the value of antibody arrays in precision oncology.
Case study 1: Plasma proteins cluster with non-responders and reveal insights on therapy resistance mechanism
In a comprehensive proteomic study of NSCLC patients on ICI therapy, Harel et al. used antibody arrays to profile 760 – 1000 plasma proteins, identifying CXCL8 and CXCL10 as markers of resistance along with age and sex. High levels of these neutrophil-recruiting chemokines were associated with poor responses and immune-suppressive pathways. Non-responders showed higher expression of neutrophil-related proteins like GRO, PLAUR and PILRA, tied to immune evasion pathways (MAPK, NF-kB, JAK-STAT) and enriched in proteins supporting cell proliferation and immunosuppression, especially in pyrimidine and adenosine metabolism. Notably, combination therapy (ICI with chemotherapy) improved response rates, suggesting that targeting these immune-suppressive and metabolic profiles could help overcome resistance.1
Case study 2: Complete response predicted by a less immunosuppressive cytokine profile
Laza-Briviesca et al. identified blood-based cytokine biomarkers predictive of complete pathological response (CPR) in stage IIIA NSCLC patients undergoing neoadjuvant chemoimmunotherapy. Baseline profiling showed that CPR patients had lower levels of neurotrophins (NT-3, b-NGF) and VEGF-D, indicating a less suppressive immune environment. After treatment, CPR patients exhibited increased immune-activating markers (4-1BB, MCSF, PARC) and decreased levels of immunosuppressive factors (Flt-3L, MPIF-1), suggesting enhanced anti-tumor responses through immune reprogramming. This study proposes cytokine profiling as a noninvasive method for predicting CPR, guiding personalized treatment strategies in NSCLC. 6
Case study 3: Combination therapy enhances immune activation
Aggressive cancers like triple-negative breast cancer and melanoma often resist treatment. Gonzalez et al. explored combining ICIs with a TLR5 agonist, CBLB502, a compound derived from bacterial flagellin that activates immune pathways via the TLR5 receptor, to enhance anti-tumor immunity in resistant cancers. Using antibody arrays and flow cytometry, they profiled 62 and 32 cytokines, respectively, to capture immune activation and suppression. Key findings showed elevated suppressive cytokines (G-CSF, CXCL5) in non-responders, while long-term responders exhibited increased IL-15, GM-CSF, IL-2, IL-13 and IFN-γ, promoting NK and T cell activation while lowering IL-10. Re-challenged survivors displayed markers of immune memory (IL-15, CXCL9, IFN-γ), suggesting durable responses. The study indicates that combining ICI therapy with TLR5 agonists could transform the tumor environment from suppression to activation, offering a targeted approach for resistant cancers through combination therapy and biomarker-driven monitoring.7
Conclusion
Antibody arrays show great potential for advancing precision medicine in ICI therapy by enabling biomarker-based personalization. By identifying key biomarkers, antibody arrays help predict patient responses, allowing clinicians to tailor therapies to individual profiles. This approach streamlines patient selection, reducing adverse effects and healthcare costs and provides insights into potential combination therapies, such as pairing ICIs with cytokine inhibitors to overcome resistance. Integrating antibody arrays into clinical workflows could make personalized ICI therapy more accessible, enhancing outcomes across diverse cancer types and contributing to precision oncology.
References
- Harel, M., et al. “Longitudinal Plasma Proteomic Profiling of Patients with Non-Small Cell Lung Cancer Undergoing Immune Checkpoint Blockade.” Journal for ImmunoTherapy of Cancer, vol. 10, 2022, e004582. https://doi.org/10.1136/jitc-2022-004582.
- Wang, M., et al. “The Role of Cytokines in Predicting the Response and Adverse Events Related to Immune Checkpoint Inhibitors.” Frontiers in Immunology, vol. 12, 2021, 670391. https://doi.org/10.3389/fimmu.2021.670391.
- Sharma, P., et al. “The Next Decade of Immune Checkpoint Therapy.” Cancer Discovery, vol. 11, no. 4, 2021, pp. 838 – 857. https://doi.org/10.1158/2159-8290.CD-20-1680.
- An, H.J., Chon, H.J., and Kim, C. “Peripheral Blood-Based Biomarkers for Immune Checkpoint Inhibitors.” International Journal of Molecular Sciences, vol. 22, no. 17, 2021, 9414. https://doi.org/10.3390/ijms22179414.
- Sharma, P., et al. “Immune Checkpoint Therapy — Current Perspectives and Future Directions.” Cell, vol. 186, 2023, pp. 1652 – 1671. https://doi.org/10.1016/j.cell.2023.03.006.
- Laza-Briviesca, R., et al. “Blood Biomarkers Associated to Complete Pathological Response on NSCLC Patients Treated with Neoadjuvant Chemoimmunotherapy.” Clinical and Translational Medicine, vol. 11, 2021, e491. https://doi.org/10.1002/ctm2.491.
- Gonzalez, C., et al. “TLR5 Agonists Enhance Anti-Tumor Immunity and Overcome Resistance to Immune Checkpoint Therapy.” Communications Biology, vol. 6, 2023, 31. https://doi.org/10.1038/s42003-022-04403-8.